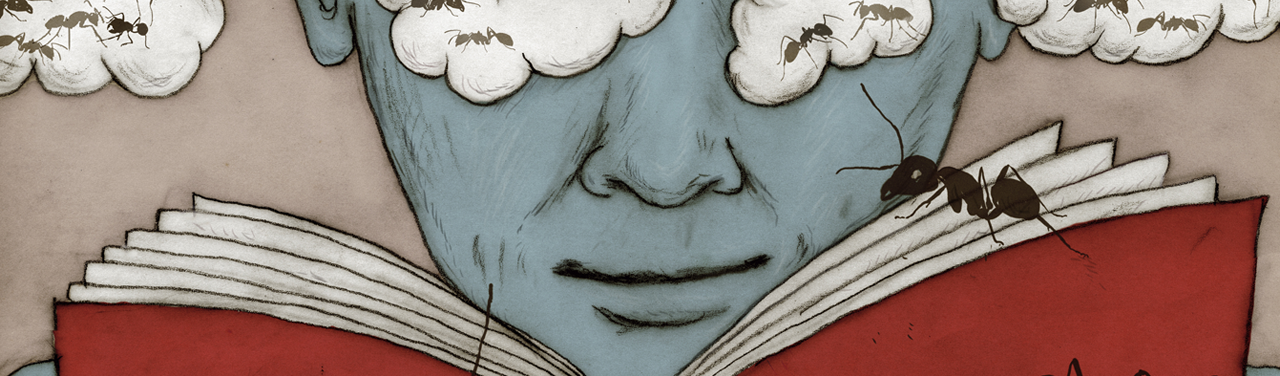
From Nautilus, Carrie Arnold studies ant colors to understand how what occurs in the synapses of neurons. Here is Mark Goldman's recollection.
“As I watched films of these ant colonies, it looked like what was happening at the synapse of neurons. Both of these systems accumulate evidence about their inputs—returning ants or incoming voltage pulses—to make their decisions about whether to generate an output—an outgoing forager or a packet of neurotransmitter,” Goldman said.The concept that connects the ants and the neurons is called feedback.
Each of the brain’s 86 billion neurons can be connected to many thousands of others. When a neuron fires, it sends a signal to nearby neurons that changes the probability that they will also fire. Some neurons are excitatory, and increase the chances that other neurons will fire. Others are inhibitory, and reduce this chance. A combination of inputs from a given neuron’s neighbors will determine if it fires. If two neurons make each other fire often, the synapse between them (a small gap across which chemical or electrical signals are passed) will strengthen, so that they can more readily provide feedback to each other.It's a fascinating article.
Ants Swarm Like Brains Think
A neuroscientist studies ant colonies to understand feedback in the brain
By Carrie Arnold | Illustration by Jonathon Rosen April 24, 2014
Deborah Gordon spent the morning of August 27 watching a group of harvester ants foraging for seeds outside the dusty town of Rodeo, N.M. Long before the first rays of sun hit the desert floor, a group of patroller ants was already on the move. Their task was to find out whether the area near the nest was free from flash floods, high winds, and predators. If they didn’t return to the nest, departing foragers would know it wasn’t safe to go search for food.
When the patrollers returned and the first foragers did leave, they scattered in all directions, hunting for the fat-laden, energy-rich seeds on which the colony depends. Other foragers waited in the entrance of the nest for the first wave to return. If lots of food were nearby, foragers would return and depart quickly, creating a massive chain reaction. If food was scarce, however, the second group of foragers might not leave the nest at all.
“It’s a brilliant system. The ants can take advantage of sudden windfalls of food but they don’t waste energy and resources if there’s nothing there,” said Gordon, who is an ecologist at Stanford University.
The behavior of each individual in the group is set by the rate at which it meets other ants and a set of basic rules. Its behavior alters that of its neighbors, which in turn affects the original ant, in a classic example of feedback. The result is astonishing, complex behavior. “Individually, an ant is dumb,” Gordon says. She gazes off into the distance and inhales sharply. “But the colony? That’s where the intelligence is.”
About 110 miles from Gordon’s offices in Palo Alto, Calif., Mark Goldman studies a different kind of complex, emergent behavior. Goldman is a neuroscientist at the University of California, Davis. For most of his life, he was never particularly interested in ants. But when he traveled to Stanford in 2012 to plan some experiments with a colleague who had recently attended one of Gordon’s talks, something clicked.
“As I watched films of these ant colonies, it looked like what was happening at the synapse of neurons. Both of these systems accumulate evidence about their inputs—returning ants or incoming voltage pulses—to make their decisions about whether to generate an output—an outgoing forager or a packet of neurotransmitter,” Goldman said. On his next trip to Stanford, he extended his stay. An unusual research collaboration had begun to coalesce: Ants would be used to study the brain, and the brain, to study ants.
Ant land: Deborah Gordon has been returning to this New Mexico landscape for years to study its ants. Carrie Arnold
Each of the brain’s 86 billion neurons can be connected to many thousands of others. When a neuron fires, it sends a signal to nearby neurons that changes the probability that they will also fire. Some neurons are excitatory, and increase the chances that other neurons will fire. Others are inhibitory, and reduce this chance. A combination of inputs from a given neuron’s neighbors will determine if it fires. If two neurons make each other fire often, the synapse between them (a small gap across which chemical or electrical signals are passed) will strengthen, so that they can more readily provide feedback to each other.
“This is where you get the saying that ‘Neurons that fire together, wire together,’ ” says Dmitri Chklovskii, a neuroscientist at the Howard Hughes Medical Institute. But what is often not appreciated about this adage is that wiring also requires the second neuron to send a message to the first that it, too, has fired.
“The only way the upstream neuron knows that the second neuron fired is that it produces a feedback spike. This helps the synapse make the decision to get stronger,” Chklovskii says. Feedback is where the similarity with ants begins. “Feedback loops are everywhere on every level. They allow the system to realize that what it used to be doing isn’t working any more, and to try something new.”
Both ants and brains actually rely on two types of feedback, held in a delicate balance: negative (or inhibitory) feedback, and positive (or excitatory) feedback. “Negative feedback tends to cause stability. Positive feedback tends to cause runaway behavior,” said Tomer Czaczkes, an ant biologist at the University of Regensburg in Germany. “These two simple rules make something very powerful.”
The foraging response to food is an example of a positive feedback loop, and familiar to anyone who has had a picnic ruined by a line of ants marching in single file toward their meal. But knowing when not to leave the nest and risk predation and dehydration may be just as important as knowing when to take advantage of a windfall of seeds. At low levels of an input (a small amount of food, for example) positive feedback dominates. At high levels of input, negative feedback dominates, helping to prevent runaway processes.
Such runaway processes can represent a significant danger to the colony. Czaczkes mentions a tale involving the South American army ant, which unlike the harvester ant, relies on pheromones for navigation. In 1936, ant biologist T.C. Schneirla watched a group of army ants fall into an “ant death spiral” as they created a pheromone trail completely around a large tree. The lead ants, Schneirla noted in a 1944 paper, discovered and followed their own pheromone trail. More and more ants joined in, following an endless positive feedback loop around the same tree, continuing for days through pouring rain until the ants began to die from exhaustion.
“Relying only on positive feedback can get you stuck like this,” said Czaczkes. He would later show that positive feedback was balanced by negative feedback in the black garden ant, and that negative feedback allowed for rapid adaptation to the environment.
Similar feedback networks are found in the brain, both at the level of individual neurons and of the whole brain. Just as the discovery of a cache of seeds by one or two harvester ants can trigger a massive exodus from the nest, the entry of a few sodium ions into a neuron can trigger a massive influx. This positive feedback raises the voltage of the neuron past a certain threshold, causing it to fire and temporarily stop the influx of sodium ions, while simultaneously letting potassium ions flood out.
The activity of individual neurons induces electromagnetic fields around the brain that can be recorded with electroencephalograms (EEGs). The EEG signal, too, is a form a positive feedback. Scientists at Yale University applied a weak electric field to a piece of ferret brain that was producing slow but regular brain waves, similar to what happens during deep sleep. This field, they found, could strengthen the existing surges of neural activity in the brain tissue, causing ripples to become tsunamis. When the scientists applied the opposite electric field to brain tissue that was experiencing regular waves of neural activity, they were able to disrupt this feedback loop.1
While these feedback processes in the brain are known to exist, they are hard to observe—much harder than analogous feedback processes in ant colonies. So Gordon and Goldman forged an ant-brain alliance. To get it started, they focused on one particular aspect of ant behavior: the number of interactions it would require for an ant to leave the nest and start foraging.Passing the baton: Returning forager ants interact with outgoing ants by touching antennae. An accumulated experience of interaction stimulates the outgoing foragers to leave the nest, analogous to the way that the accumulated input from other neurons stimulates a neuron to fire. Deborah Gordon, Stanford UniversityIn August 2013, Gordon returned to the same desert scrubland straddling the border of Arizona and New Mexico that she has visited each summer for 30 years. Armed with a map and a clipboard, she strode over the packed dirt and patches of thick grass to begin her experiments. As she walked past harvester ant colonies, she greeted them like old friends. “Happy birthday, Colony 330. You made it another year,” she said as she scribbled a note on a piece of paper, never breaking stride.
Flanked by a group of undergrads and Ph.D. students, Gordon arrived at a nest she had marked 889 with a piece of rusty wire and a stamped metal tag. Everyone set down their equipment, which included everything from large plastic buckets to umbrellas to peanut butter. Gordon removed a roll of blue duct tape decorated with pictures of SpongeBob SquarePants. The students immediately began tucking shirts into pants and jean legs into socks. Then, they carefully wrapped SpongeBob tape around ankles, wrists, and waist—anywhere the ants might be able to deliver a nasty bite. Neuroscience research had never looked quite like this before.
After taping their clothes, the students set up two video cameras. One was aimed at the opening of the nest, a hole in the ground that looks like it could have been drilled by a pencil. Another was aimed at a square about three feet from the nest entrance, measuring about one foot on each side, and with rocks at the corners. Armed with a plastic spoon filched from the cafeteria of the Southwestern Research Center that morning, Gordon began to enlarge the tiny nest entrance. Then cameras started rolling.
For 20 minutes, the group recorded the harvester ants going about life as usual, leaving the nest in search of food and bringing seeds back home. Then, Gordon focused her attention on the square. Like a Cordon Bleu chef pinching a bit of salt, Gordon expertly grabbed the ants in the square between thumb and forefinger, dropping them into a plastic bucket. The cameras kept rolling as the normally bustling ant entrance went eerily still.
“The ants aren’t getting feedback from their nestmates,” she explained. “No one is coming in, so the ants inside aren’t getting the signal to leave.”
Gordon and her team found that it took just three seconds for harvester ants to start leaving the nest faster, after their nestmates began returning in droves with food.2 The opposite reaction was much slower: If foragers didn’t return for four to five minutes, ants near the entrance fled deeper underground. The team also measured how long it took the colony to return to normal after being disrupted.
Numbers like these are precious information for Goldman. Like ants, neurons also have first and second responders. When a neuron fires, it releases neurotransmitters that are housed in containers called vesicles. One pool of vesicles, called the “readily releasable pool,” lives right at the cell membrane and is the first to be released. The vesicles in this pool, however, are few in number and can quickly be depleted. If firing rates continue at a moderate level, a second pool of vesicles called the “recycling pool” is brought into action, and very intense rates will summon a third pool called the “reserve pool.”
A returning forager is like an incoming action potential; a potential forager in the ant colony is like a synaptic vesicle. These potential foragers also appear to be arranged according to their proximity to the tunnel entrance. Those further back are similar to the recycling and reserve pools. Just as a potential forager has to decide whether to leave the nest in search of food, the synapse has to decide whether to release a vesicle.
Goldman believes he can use ant data to understand the foraging behavior of the desert ants and the ways in which it is similar to or different from vesicle recycling. To understand ant foraging behavior, he is using a mathematical construct called a stochastic accumulator. This model sums the inputs that the ants receive inside a certain time window, in a way that takes into account the inherent randomness of the environment. In synapses, the chemical signals that trigger ant foraging behavior are replaced by electrical signals that trigger the release of neurotransmitter, and the leave/stay decision of the ant is replaced by the release/don’t release decision of the synapse.
For her part, Gordon is hoping for more precise mathematical models of ant behavior. She hopes it will help her understand how differing responses to interactions at the level of individual ants may lead to differences among colonies. For example, colonies that forage less when conditions are dry are more likely to produce daughter colonies. This adaptation may result from ants requiring more interactions with their nestmates before leaving the nest to forage. “Gathering food can be really risky,” Gordon said. “It actually benefits the colony to show restraint, because they’re not wasting resources.”
In the months since her return from New Mexico, Gordon has spent many of her days watching her newly collected videos. Together with her team of postdocs, graduate students and undergrads, she counts the ants as they swarm around the colony entrance. Each ant arrival receives a sharp click on the stopwatch-like counter the students use, so that the analysis sounds like a machine gun battle in the corner of her Stanford laboratory. With each click, one species moves closer to understanding another—and itself.
~ Carrie Arnold is a freelance science writer living in Virginia. She has covered many aspects of the living world for Scientific American, Discover, New Scientist, NOVA, and other publications.
References
1. Fröhilch, F. & McCormick, D.A. Endogenous electric fields may guide neocortical network activity. Neuron 67 (1), 129-143 (2010).
2. Pinter-Wollman, N., et al. Harvester ants use interactions to regulate forager activation and availability. Animal Behaviour (2013). Retrieved from http://dx.doi.org/10.1016/j.anbehav.2013.05.012.
Bagaimana Menarikkan Article Pada Hari Ini . BLUE.Jangan Lupa Datang Lagi Untuk Membaca Article Yang lebih Menarik Pada Masa Akan Datang/
Posting Komentar